53 Memory and the Brain
Learning Objectives
By the end of this section, you will be able to:
- Describe the role of brain regions in different types of memory
- Describe how neurons store memory
- Discuss the role of specific neurotransmitters in memory
Where are memories stored? Karl Lashley began exploring this problem about 100 years ago, by making lesions in the brains of non-human animals. He was searching for evidence of the engram: the group of neurons that serve as a physical memory trace (Josselyn, 2010). First, Lashley trained rats to find their way through a maze, and then he created lesions in the cerebral cortex to try and erase the memory trace that the rats had of the maze. He didn’t find evidence of the engram since the rats were still able to find their way, regardless of the size or location of the lesion. Based on these findings, he formulated the equipotentiality hypothesis: if part of one area of the brain involved in memory is damaged, another part of the same area can take over that memory function (Lashley, 1950). Although Lashley failed to find evidence of the engram, he is considered a pioneer in the field of neuroscience.
Since Lashley’s research, other scientists have been able to look more closely at the role of the brain in memory. They have argued that memory processes recruit distinct brain areas, and specific neurons can be recognized for their involvement in forming memories. Although the entire brain is involved in memory in some way or another, we’ll focus on some key players: the hippocampus, amygdala, cerebellum, and prefrontal cortex (Figure M.21).
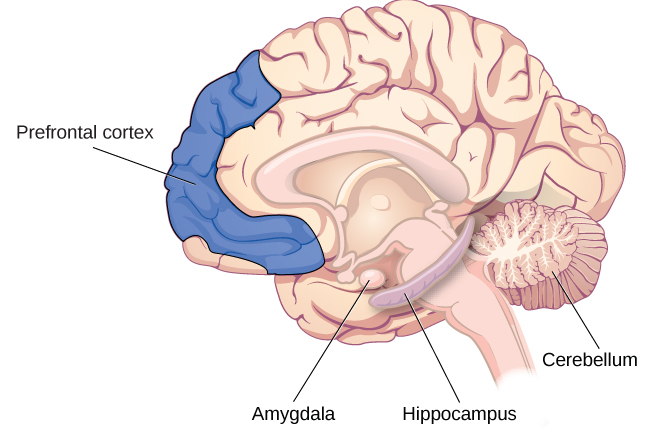
Hippocampus
Experimental surgeries conducted on humans in the 1900s have taught us that memory is indeed tied to discrete brain regions. One famous example is Patient HM, who underwent a temporal lobectomy and had his anterior hippocampus, parahippocampal gyrus, and amygdala removed (for a refresher on HM, check out the Intro section). After his surgery, he couldn’t create any new explicit memories but still had his early-life memories and the ability to learn new procedural tasks (Milner, 2005; Scoville, 1954). Thanks to HM, we have learned that the medial temporal lobes are involved in explicit memory formation but are not involved in implicit learning or memory storage.
Much of what we know about the role of the brain in human memory is the result of studying people with severe brain injuries. However, it is difficult to pinpoint the exact function of a brain region when multiple areas are damaged. Researchers are better able to make precise lesions in non-human animals to understand the role of specific brain areas. However, this presents a challenge: you can’t ask a rat to recall facts about their childhood like you can with HM Instead, researchers use tasks like mazes in combination with lesions or sham surgeries to test memory, such as the Morris water maze. A sham surgery is when the control group undergoes an imitation of the surgery but does not receive the procedure itself. Researchers first train animals, usually rats or mice, to swim to hidden underwater platforms. Then, they’re lesioned in specific areas and retested to see if they can remember the platform’s location. They have no issues finding the platform if they learned the location before hippocampal damage but struggle to learn the platform’s location if lesioned before training. This shows that the hippocampus is critical in spatial memory formation (Steffenach et al., 2002) (Figure M.22).
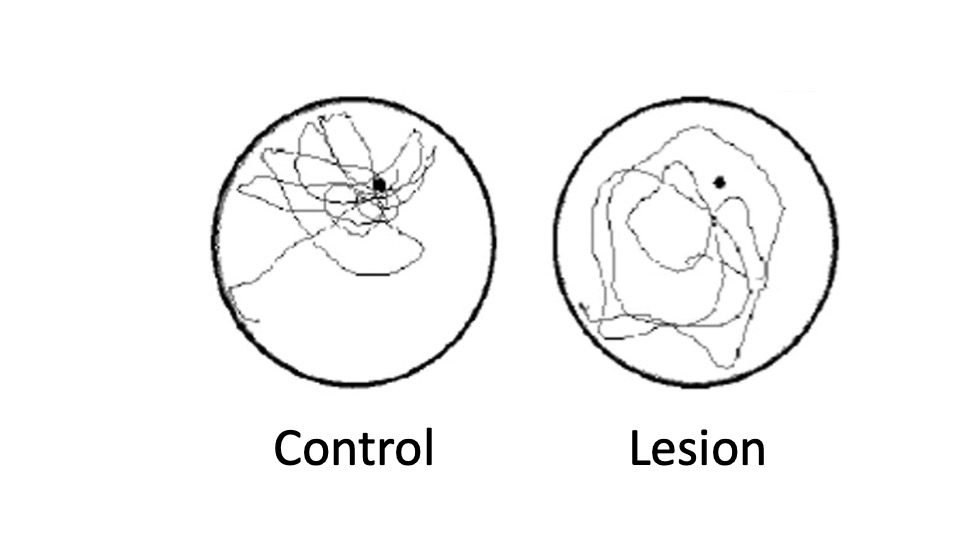
The opportunity to study people like HM is rare since it’s unethical to inflict brain damage on humans solely for the purposes of research. Instead, researchers use neuroimaging and spatial memory tasks as techniques to understand the brain. For example, to check that water mazes used with rodents can model the same systems in humans, a research team from McGill University conducted a virtual water maze experiment on ten human patients who had undergone unilateral hippocampal removal surgery to treat incurable epilepsy, like HM. Two control groups were used; healthy people with no surgery history and those who underwent tumour removal surgery. The tumour removal group had normal, non-damaged hippocampi. First, all volunteers underwent “hidden platform” training, where they could not see the platform and had to practice trying to find it. Then they went through “visible platform training,” where they could see the platform and had to swim toward it. In the visible condition, everyone swam toward the platform equally well. However, when the platform was hidden, the hippocampal removal group were slower to find the platform and spent less time in the correct quadrant of the pool (Figure M.23) (Astur et al., 2002).
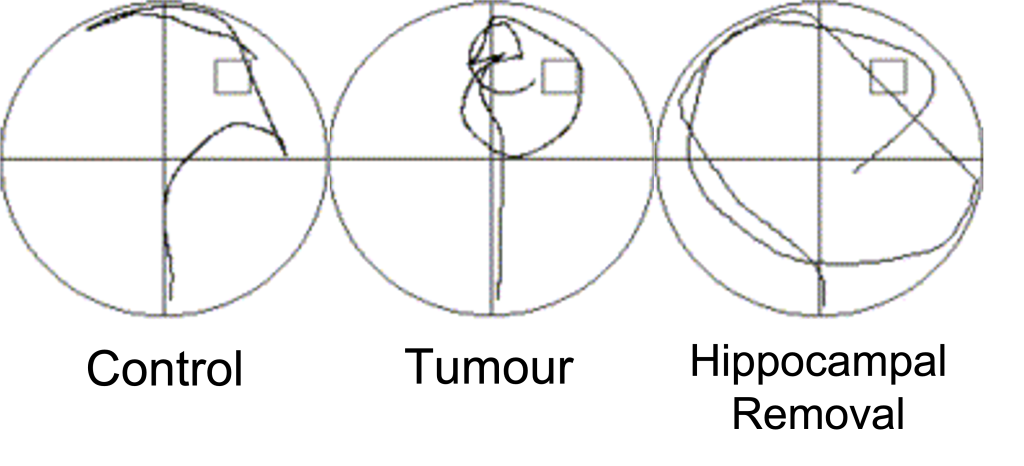
Substances like cannabis affect the hippocampus and our ability to form spatial memories. For example, long-term, regular cannabis usage in humans has been shown to cause a decrease in hippocampus size, volume, and integrity (Yucel et al., 2016). In addition, studies in rats have shown that THC, the psychoactive ingredient in cannabis, produces memory impairments similar to the effects of hippocampal lesions, causing poorer performance in tasks that require spatial memory (Hampson & Deadwyler, 1998).
TRICKY TOPIC: HIPPOCAMPUS & MEMORY
If the video above does not load, click here: https://youtu.be/W7AgZzS7Iuc
Amygdala
Why are some types of memory easier to remember than others? For example, why are emotionally arousing memories like your first kiss or your pet passing away easier to remember than your breakfast last week? Emotions provide a “boost” to our memory, but how? Neuroimaging studies show that the amygdala becomes highly active when viewing emotionally stimulating events. For example, one study had participants watch emotionally negative film clips, such as violent crime, and emotionally neutral ones, like court proceedings. Recall was better for emotional films and was positively correlated with amygdala activation, as measured by PET imaging (Cahill et al., 1996) (Figure M.24).
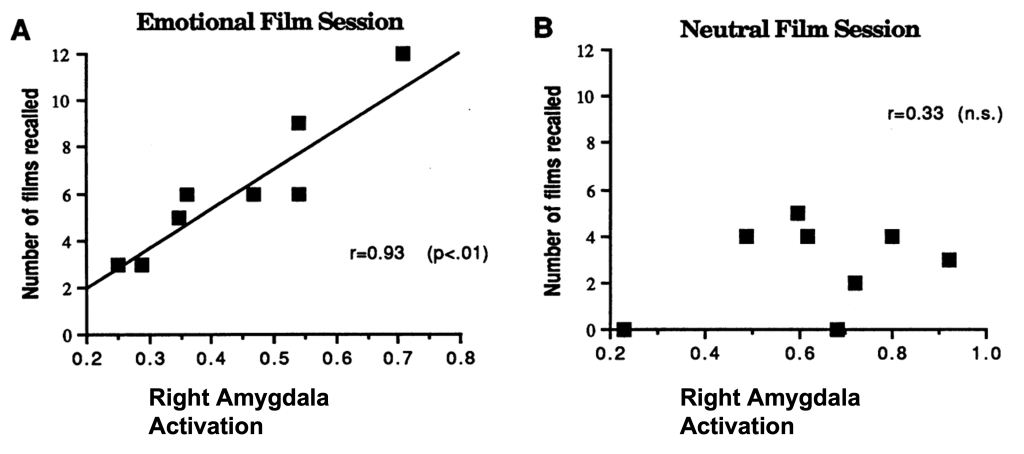
The Cerebellum and Prefrontal Cortex
The hippocampus is important for laying down certain types of explicit memories, but its absence doesn’t affect the ability to create implicit memories, such as procedural memory, motor learning, and classical conditioning, thanks to your cerebellum (Figure M.21). For example, one classical conditioning experiment involves training subjects to blink when given a puff of air to the eye. However, when researchers damaged the cerebellum of rabbits, they discovered that they were not able to learn the conditioned eye-blink response (Steinmetz, 1999; Green & Woodruff-Pak, 2000).
Other researchers have used brain scans, including positron emission tomography (PET) scans, to learn how people process and retain information. From these studies, it seems the prefrontal cortex is involved. For example, in one study, participants had to complete two different tasks: either looking for the letter a in words (considered a perceptual task) or categorizing a noun as either living or non-living (considered a semantic task) (Kapur et al., 1994). Participants were then asked which words they had previously seen. Recall was much better for the semantic task than for the perceptual task. According to PET scans, there was much more activation in the left inferior prefrontal cortex in the semantic task. In another study, encoding was associated with left frontal activity, while information retrieval was associated with the right frontal region (Craik et al., 1999).
TRICKY TOPIC: BIOLOGICAL BASIS OF MEMORY
If the video above does not load, click here: https://youtu.be/_LIiYy-BRes
For a full transcript of this video, click here