4.3 The Endomembrane System
KEY CONCEPTS
By the end of this section, you will be able to do the following:
- Compare the roles of vesicles and vacuoles within the endomembrane system
- Recognize the structure and describe the function of the endoplasmic reticulum (smooth and rough), Golgi apparatus, lysosomes, and the central vacuole of plants
- Explain which components of the endomembrane system are physically linked (shared a membrane) versus linked by vesicles
- Evaluate how different endomembrane system components work together to modify and transport macromolecules within and out of eukaryotic cells
- Give examples of how the abundance or structure of each endomembrane system component varies among different cell types
The endomembrane system (endo = “within”) is a group of membranes and organelles in eukaryotic cells that works together to synthesize, modify, package, store, transport, and digest macromolecules like lipids and proteins within the cell. Macromolecules can also be transported out of the cell via the endomembrane system. This system includes the nuclear envelope, the endoplasmic reticulum, Golgi apparatus, lysosomes, central vacuole, and vesicles, some of which are depicted in Figure 4.9. Although not technically within the cell, the plasma membrane is included in the endomembrane system because, as you will see, it interacts with the endomembranous organelles. The endomembrane system does not include mitochondria, chloroplasts, or peroxisomes.
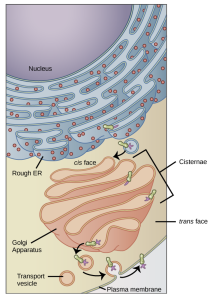
Link to Learning
Vesicles and Vacuoles
Vesicles and vacuoles are membrane-bound sacs that function in storage and transport of materials within cells. Vesicles typically range from 20-50 μm in diameter, while vacuoles tend to be larger and can sometimes fill most of the cell’s volume. Other than the fact that vacuoles are somewhat larger than vesicles, there are very few distinctions between them. Vesicle membranes can fuse with either the plasma membrane or the membranes of various organelles in the endomembrane system. The term “vacuole” is used to describe a diverse array of both temporary structures (e.g., the “food vacuole” that results from phagocytosis) and organelles with lysosome-like function (e.g., lytic vacuole) or storage functions. The central vacuole in plant cells is the largest vacuole; it is a permanent structure and is described in further detail below. Generally, in this section, we use the term “vacuole” to refer to the central vacuole of plant cells.
The Endoplasmic Reticulum
The endoplasmic reticulum (ER; Figure 4.10 and 4.11) is a series of interconnected membranous sacs and tubules. The flattened membrane-bound sacs and tubes of the ER are called cisternae. Within the cisternae lies an internal space that is called the lumen or cisternal space. The ER’s membrane, which is a phospholipid bilayer embedded with proteins, is continuous with the outer membrane of the nuclear envelope (Figure 4.10). The size of ER can vary, but typically the lumen of the rough ER is 20-30 nm wide, while the smooth ER lumen is 30-60 nm wide. Depending on a cell’s function, ER membrane may comprise up to half of the total amount of membrane within a cell! The components of the ER collectively perform many functions (described below), which take place in either the rough ER or the smooth ER.
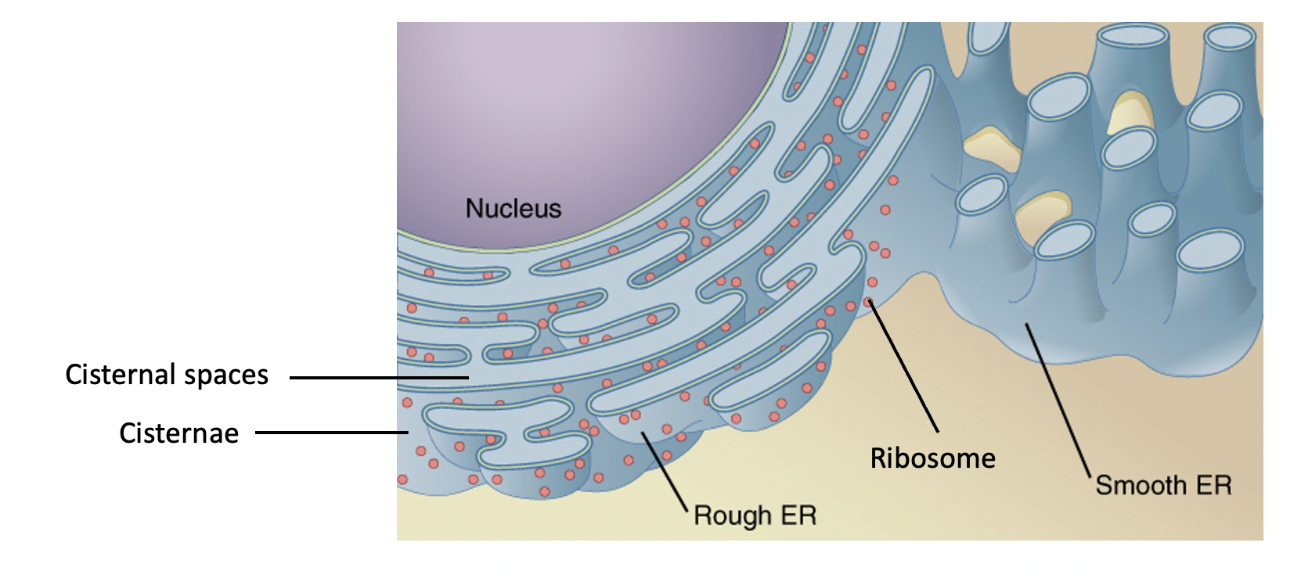
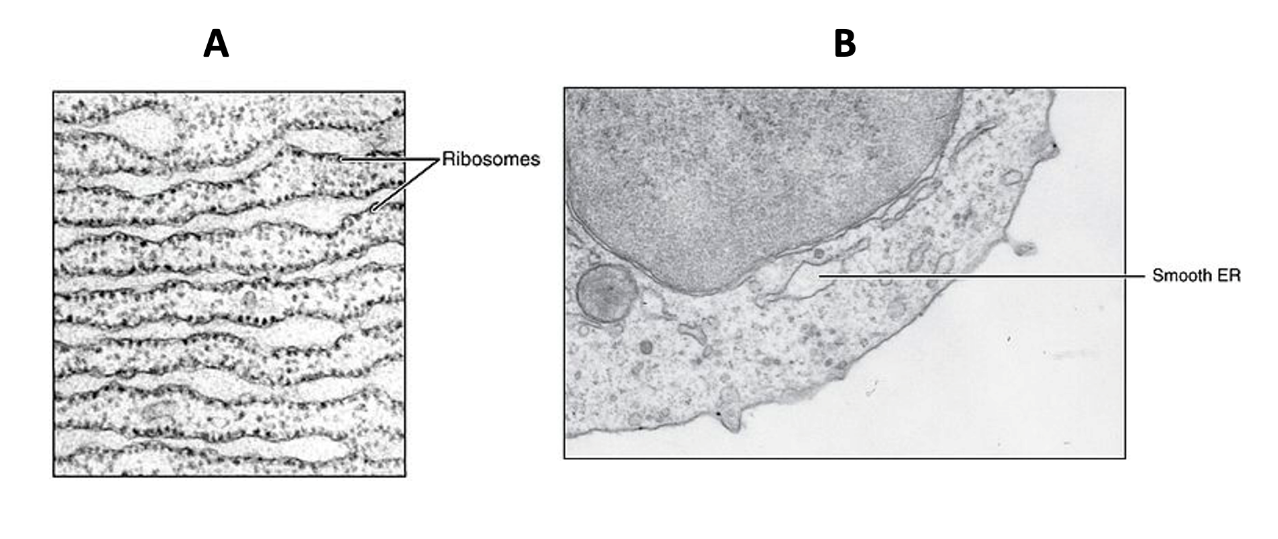
Rough ER
Scientists have named the rough endoplasmic reticulum (RER) as such because the ribosomes attached to its cytoplasmic surface give it a studded (rough) appearance when viewed through an electron microscope (Figure 4.11). The RER has multiple roles related to proteins, including protein synthesis, folding, modification, and transport to other places in the cell.
Ribosomes on the RER surface transfer their newly-synthesized proteins into the RER lumen, where they undergo structural modifications, such as folding or acquiring side chains. Most of these proteins will leave the RER via vesicles, which transport the proteins to the Golgi on the way to their final destination. Most proteins synthesized by ribosomes on the RER will be secreted outside the cell or end up in a membrane (e.g., the plasma membrane). Some examples of secreted proteins from animal cells are digestive enzymes, antibodies, and hormones. Examples of membrane proteins include transmembrane protein channels that are involved in transmembrane transport (see Chapter 5). Since the RER is engaged in processing proteins destined for secretion, the RER is abundant in cells that secrete proteins like human pancreatic cells.
Human Connection: Maintaining Homeostasis
One of the protein hormones that can be produced by the RER is insulin, which is important for regulating blood glucose levels. Beta cells in the human pancreas have a large RER to support the function of synthesizing and secreting insulin to regulate our blood sugar. Membrane proteins synthesized by the RER also play important roles in homeostasis, such as sodium-potassium ATPase, which is responsible for maintaining the resting membrane potential. All animal cells must make this protein and embed it in the plasma membrane.
Smooth ER
The smooth endoplasmic reticulum (SER) is continuous with the RER but has few or no ribosomes on its cytoplasmic surface (Figure 4.11). Apart from lacking ribosomes, the structure of the SER is similar to the RER; it has cisternae (arranged into tubules) that are enclosed by a phospholipid membrane, creating an the inner lumen space. SER functions include carbohydrate metabolism, lipid (including steroid hormone) synthesis, detoxification of some chemicals, and calcium ion storage. Vesicles that leave the SER naturally contain any membrane lipids (e.g., phospholipids) that were synthesized in the SER, but steroid lipids leave the SER via simple diffusion across the SER membrane. The SER does not perform all of these functions in all cell types. For example, human liver cells have SER that engage in drug detoxification. Human muscle cells have a specialized SER (the sarcoplasmic reticulum) that stores calcium ions that are needed to trigger the muscle cells’ coordinated contractions. Some human endocrine cells have SER specialized for steroid hormone synthesis.
The Golgi Apparatus
We have already mentioned that vesicles can bud from the ER and transport their contents elsewhere, but where do the vesicles go? Before reaching their final destination, the contents of the transport vesicles still need sorting, packaging, and tagging so that they end up in the right place, a major function of the Golgi apparatus (also called the Golgi body). In plant cells, the Golgi apparatus has the additional role of synthesizing polysaccharides, some of which are incorporated into the cell wall. This organelle consists of a series of flattened membranous sacs (Figure 4.12). The membrane bound flattened stacks of the Golgi are called cisternae. The size and number of Golgi apparatuses varies among cells, but each Golgi apparatus is approximately 0.5 μm wide (Figure 4.12). Note that “Golgi” is always capitalized because the organelle is named after a person.
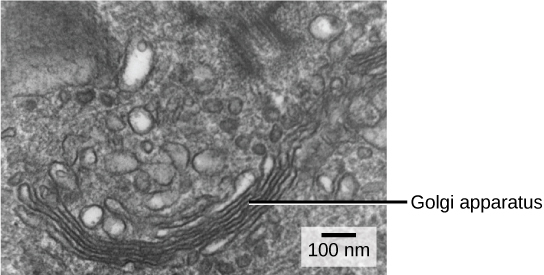
The side of the Golgi apparatus that is closer to the ER is called the cis face. The opposite side (furthest from the ER) is the trans face. The part in between the cis and trans face consists of the medial stack(s). The transport vesicles that formed from the ER travel to the cis face, fuse with it, and empty their contents into the Golgi apparatus’ lumen. As the proteins and lipids travel through the Golgi, they undergo further modifications that allow them to be sorted to other parts of the cells or the extracellular space. The most frequent modification is glycosylation: the process of adding short sugar molecule chains to other molecules like proteins. Finally, the modified and tagged proteins and lipids are packaged into vesicles that bud from the Golgi’s trans face. While some of these vesicles deposit their contents into other organelles of the endomembrane system where they will be used, secretory vesicles fuse with the plasma membrane and release their contents outside the cell (Figure 4.9).
Lysosomes
Animal cells have a set of organelles that most plant cells do not: lysosomes. The lysosomes are small organelles that are approximately 60 nm-1 μm in size and they are the cell’s “garbage disposal.” The lysosome’s size can increase or decrease depending on the contents brought to it. They function to digest macromolecules, non-functional organelles, and other large cellular components. In plant and fungal cells, this intracellular digestion takes place in acidic vacuoles instead, including the central vacuole of plant cells.
The enzymes that macromolecules within the lysosomes are called acid hydrolases or hydrolytic enzymes. As their name implies, these enzymes function best in acidic environments (low pH) and function to catalyze hydrolysis reactions (breaking bonds with the help of water). These enzymes are active at a much lower pH than those enzymes of the cytoplasm. The pH within lysosomes is more acidic than the cytoplasm’s pH, allowing optimal function of the hydrolytic enzymes. The acidic environment inside the lysosomes is maintained by proton pumps in the lysosomal membrane, which pump hydrogen ions from the cytosol and into the lysosomes. One advantage of the hydrolytic enzymes only being active at a low pH is that if any of these enzymes were to escape into the cytosol, they would become denatured (due to the higher pH environment of the cytosol) and unable to accidentally digest normal, functional components of the cytoplasm. Many reactions that take place in the cytoplasm could not occur at a low pH, so again, the advantage of compartmentalizing the eukaryotic cell into organelles is apparent.
Lysosomes can also use their enzymes to destroy pathogens (disease-causing organisms) that might enter the cell. A good example of this occurs in macrophages, a group of white blood cells which are part of your body’s immune system. In a process called phagocytosis, a section of the macrophage’s plasma membrane invaginates (folds in) and engulfs a pathogen (see Chapter 5 for more details on phagocytosis). The invaginated section, with the pathogen inside, pinches itself off from the plasma membrane and becomes a vesicle. The vesicle then fuses with a lysosome and the lysosome’s enzymes then destroy the pathogen (Figure 4.12).
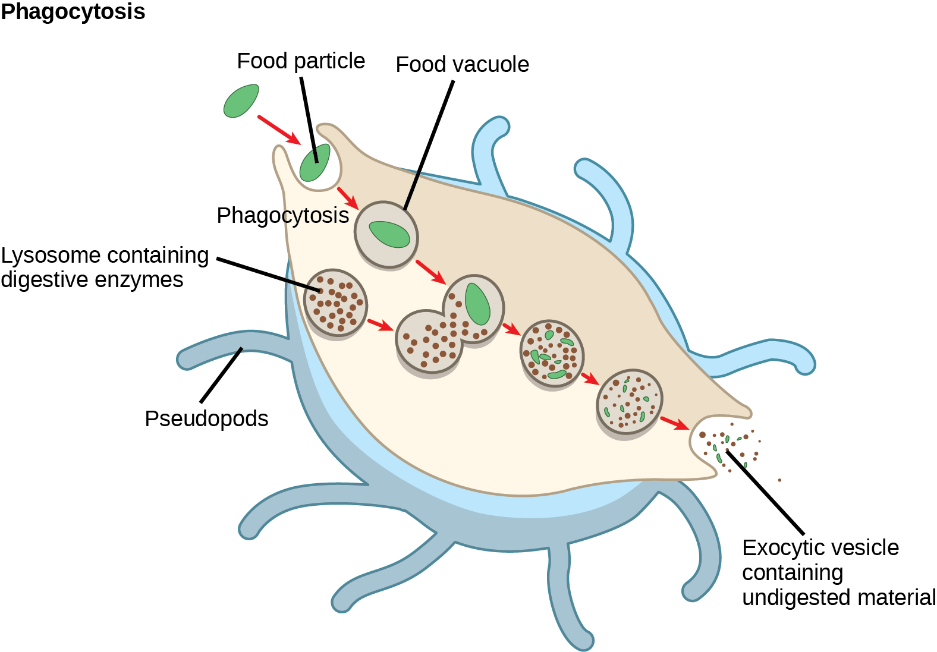
The Central Vacuole
The central vacuole is the largest organelle of most plant cells, often they are bigger than the nucleus, and occupying most of the cell’s volume (Figure 4.14). Similar to lysosomes in animal cells, the central vacuole contains hydrolytic enzymes that degrade materials. The central vacuole also helps to regulate the pH of the cytosol via proton pumps. In addition, the central vacuole plays a key role in regulating the cell’s concentration of water in changing environmental conditions. Have you ever noticed that if you forget to water a plant for a few days, it wilts? That’s because as the water concentration in the soil becomes lower than the water concentration in the plant, water moves out of the central vacuoles and cytoplasm. As the central vacuole shrinks, it leaves the cell wall unsupported. This loss of support to the plant’s cell walls results in a wilted appearance at the whole-plant level. The central vacuole also supports the cell’s expansion. When the central vacuole holds more water, the cell becomes larger without having to invest considerable energy in synthesizing new cytoplasm. This force exerted by the vacuole against the cell wall is called turgor pressure. Finally, the central vacuole can store other molecules, depending on the type of cell. Some other functions of the central vacuole are the storage of proteins, nutrients, wastes, pigments, toxins and protective compounds.
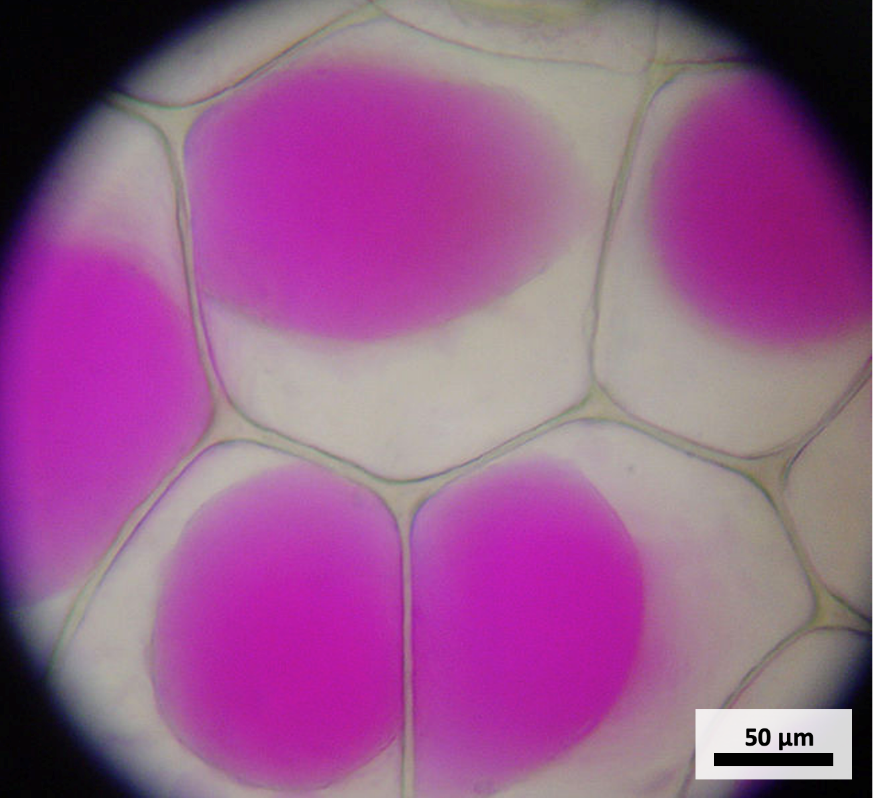
Connecting the Dots
The organelles of the endomembrane system are all connected; the organelles are either directly continuous with one another (e.g., the nuclear envelope and ER membrane), or they are connected via vesicles (Figure 4.9). The RER synthesizes proteins, and the SER synthesizes lipids, hormones and steroids. These products are packaged into vesicles and brought to the cis face of the Golgi for modification. The Golgi acts as the post office of the cell, to sort and package macromolecules and other products, to their destination in or out of the cell. Some proteins are modified by the Golgi include hydrolytic enzymes that leave the Golgi’s trans face in vesicles, and those vesicles fuse with lysosomes (animal cells) or a central vacuole (plant cells) to deliver the enzymes to the interior of the lysosomes. Proteins destined for secretion will get packaged into secretory vesicles to fuse with the plasma membrane, releasing those proteins into the extracellular environment. Newly synthesized phospholipids and integral membrane proteins are incorporated into the membranes of vesicles that bud from the Golgi’s trans face, and these phospholipids will join the membrane with which the vesicle fuses. Each organelle plays a unique and valuable role in maintaining the normal everyday activities of the cell.