5.3 Passive Transmembrane Transport
KEY CONCEPTS
By the end of this section, you will be able to do the following:
- Compare and contrast the mechanisms of simple and facilitated diffusion, and which types of solutes can move across membrane in these ways.
- Evaluate the factors that affect the rates of simple and facilitated diffusion.
- Understand the difference between osmolarity and tonicity, and their related terms (e.g., hyperosmotic, hypertonic).
- Explain how osmolarity and tonicity impact osmosis in cells.
In passive transport, solutes move across a membrane via diffusion, meaning the solutes move down (with) their concentration gradient (neutral solutes) or electrochemical gradient (charged solutes). All solutes that can directly cross the membrane (without a protein channel or carrier) can engage in passive transmembrane transport – a form of transport that requires no direct energy input from the cell. In addition, many charged and polar solutes can cross membranes via passive transport if the appropriate protein channels or carriers exist in that membrane. We will discuss several types of passive transport in this section, including simple diffusion, facilitated diffusion, and osmosis.
Simple Diffusion
Simple diffusion is a type of passive transmembrane transport in which solutes pass directly through the phospholipid bilayer from an area of high solute concentration to an area of low solute concentration until dynamic equilibrium is reached. Many gases, hydrophobic molecules, and some small very small polar molecules (e.g., water) freely move across a membrane down their concentration gradients (review Figure 5.14).
Several factors affect the rate of simple diffusion. First is the extent of the concentration gradient. The greater the difference in concentration, the more rapid the diffusion. The closer the distribution of the material gets to equilibrium, the slower the diffusion rate. Second is the mass of the solutes diffusing. Heavier molecules move more slowly; therefore, they diffuse more slowly. Third is the solubility of the substance. Nonpolar or lipid-soluble materials pass through membranes more easily than polar materials, allowing a faster diffusion rate. The final factor is the surface area and thickness of the membrane. Greater surface area increases the diffusion rate, whereas a thicker membrane reduces it.
Facilitated Diffusion
In facilitated diffusion, materials diffuse across the membrane with the help of transmembrane proteins such as the channels and carrier proteins discussed in Chapter 5.2. The types of solutes that cross membranes via facilitated diffusion included polar molecules (e.g., sugars, amino acids) and charged particles (ions) that are usually repelled by the cell membrane’s hydrophobic core (review Figure 5.14). Some molecules like water (H2O) can actually cross membranes via simple or facilitated diffusion if the appropriate channels (e.g., aquaporins) are present in that membrane. Transport proteins shield these solutes or other molecules from the hydrophobic inner portion of the membrane, facilitating their diffusion across a membrane.
Glucose is an important source of energy for all cells. However, glucose cannot simply diffuse through the plasma membrane due to its size and polar (hydrophilic) properties. Consequently, glucose must find another way through the membrane, and it does so through facilitated diffusion. For example, human red blood cells (RBCs) constantly use glucose; therefore, they tend to have a very low concentration of glucose in their cytosol. This sets up a concentration gradient, where the concentration of glucose is higher outside than inside RBCs. Thus, glucose can move down its concentration gradient from the blood (outside the cell) into the cytosol of RBCs via protein channels (Figure 5.20).
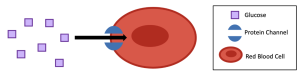
Many of the factors that influence rates of simple diffusion also influence rates of facilitated diffusion. However, membrane surface area does not impact rates of facilitated diffusion, but rather the abundance of open/active channels or carrier proteins. Cells can increase rates of facilitated transport by either embedding more appropriate transport proteins into a cell membrane, or by opening gated channel proteins that already exist in the membrane.
Link to Learning
Osmosis
Osmosis is a special case of diffusion that applies only to the movement of free water molecules across a semipermeable membrane. Water moves via osmosis according to the water’s concentration gradient, which is inversely proportional to the gradient of solutes across that membrane. That is, water diffuses from areas of low solute concentration (high water concentration) to areas to high solute concentration (low water concentration).
Let’s look at an example. Imagine a beaker with a semipermeable membrane separating the two sides or halves (Figure 5.21). On both sides of the membrane the water level is the same, but there are different solute concentrations. In this scenario, the solute cannot cross the membrane; otherwise, the solute crossing the membrane would balance concentrations on each side. If the solution’s volume on both sides of the membrane is the same, but the solute’s concentrations are different, then there are different amounts of water, the solvent, on either side of the membrane. Water has a concentration gradient in this system. Thus, water will diffuse down its concentration gradient, crossing the membrane to the side where it is less concentrated. This diffusion of water through the membrane—osmosis—will continue until the water’s concentration gradient (and the solute concentration gradient) is dissipated.
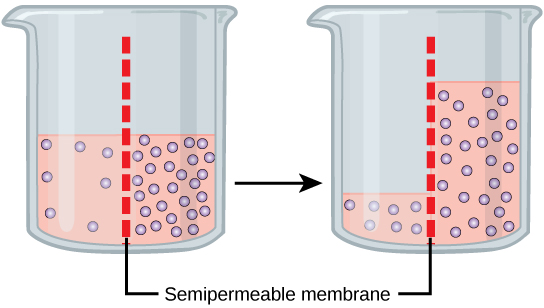
Osmolarity
One very useful principle for understanding osmosis is osmolarity. Osmolarity describes the solution’s total solute concentration. A solution with low osmolarity has low solute concentration and therefore a greater number of water molecules relative to the number of solute particles. A solution with high osmolarity has high solute concentration, and therefore has fewer water molecules with respect to solute particles. All solutes dissolved in a fluid contribute to its osmolarity. The osmolarity of two solutions on either side of a semipermeable membrane will impact the movement of water (osmosis) across that membrane.
The terms hyperosmotic, hypoosmotic, and isosmotic are used to describe the relative osmolarities between solutions. When comparing solutions that have different osmolarities, the solution with the higher osmolarity is said to be hyperosmotic (“hyper” = more/above) to the other, and the solution with lower osmolarity is said to be hypoosmotic (“hypo” = less/below). If two solutions have the same osmolarity, they are said to be isosmotic (“iso” = equal). In a situation in which a membrane permeable to water, but not to the solute, separates two solutions of different osmolarities, water will move from the side of the membrane with lower osmolarity (and more water) to the side with higher osmolarity (and less water). This effect makes sense if you remember that the solutes cannot move across the membrane, and thus the only component in the system that can move—the water—moves along its own concentration gradient.
Osmosis proceeds constantly in living systems. Water can move directly across cellular membranes (simple diffusion) even though it is polar. This is because it is very small. Many cells also contain channels in their cell membrane called aquaporins (Figure 5.22), which allow water to move across the membrane more quickly via facilitated diffusion. Both of these (simple and facilitated diffusion of water) contribute to osmosis.
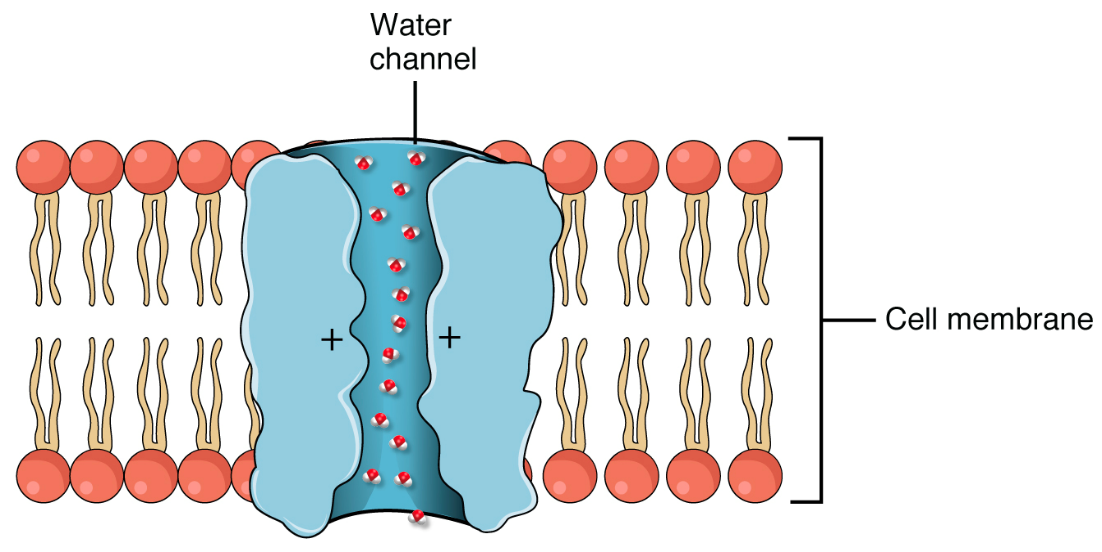
Tonicity
Tonicity describes how an extracellular solution can change a cell’s volume by affecting osmosis. A solution’s tonicity often directly correlates with the solution’s osmolarity; however, tonicity is used specifically to compare the osmolarity of an extracellular fluid to that of a cell’s cytosol. Conversely, osmolarity can be used to describe any fluid (including in non-biological systems).
We use three terms—hypotonic, hypertonic, and isotonic—to relate the osmolarity of the extracellular fluid to the osmolarity inside a cell, with consequences for osmosis (Figure 5.23). In a hypotonic situation, the extracellular fluid has lower osmolarity than the fluid inside the cell, and water enters the cell via osmosis. In a hypertonic solution, the extracellular fluid has a higher osmolarity than the cell’s cytosol, and water will leave the cell via osmosis. In an isotonic solution, the extracellular fluid has the same osmolarity as the cell’s interior, so there should be no net movement of water into or out of the cell.
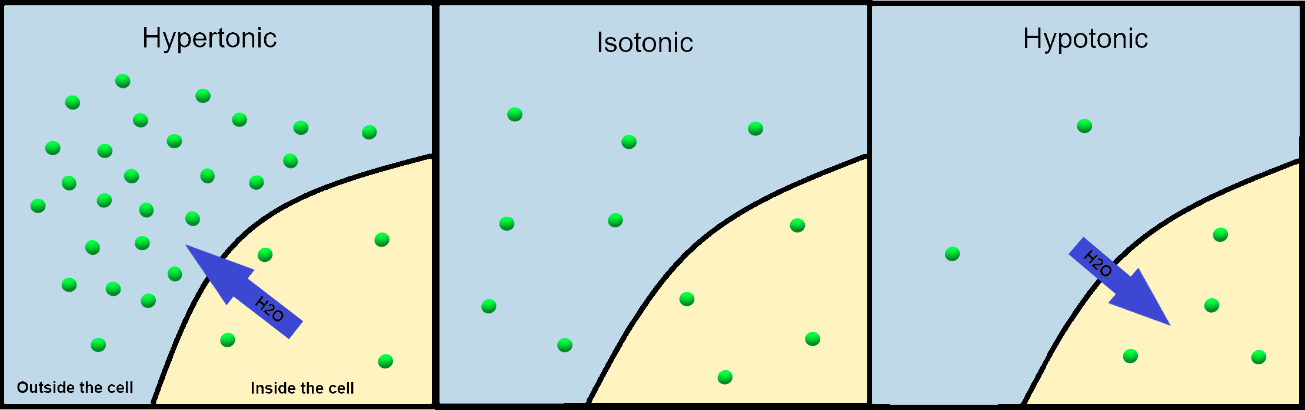
Lots of organisms work hard to control the osmolarity in their cells and other fluids, a process called osmoregulation. For example, in humans the osmolarity of our blood is tightly controlled to be similar to that of the cytosol of red blood cells. This prevents these cells from shrinking or bursting due to osmosis. Any drugs that are administered intravenously (into the blood) must be administered in a liquid that is isotonic to blood cells as well. Some organisms, such as plants, fungi, bacteria, and some protists, have cell walls that surround the plasma membrane and prevent cell lysis in a hypotonic solution. The plasma membrane can only expand to the cell wall’s limit, so the cell will not lyse (Figure 5.24). In fact, you can use this knowledge to keep your vegetables crispy: if you place a stalk of celery (or a better vegetable) in glass of tap water, the relatively hypotonic environment of the tap water will keep water moving into the celery cells, but shouldn’t cause damage to the cells. Other organisms such as paramecia and amoebas, which are protists that lack cell walls, have contractile vacuoles. As the cell takes on water from its hypotonic environment, the contractile vacuole collects excess water from the cell and pumps it out, keeping the cell from lysing (Figure 5.25).
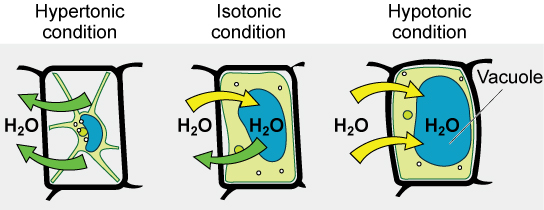
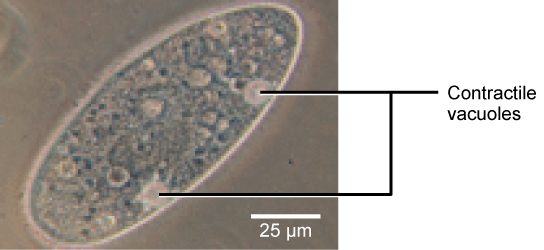